
CQE PI Feature – Soonwon Choi
Featured in QSEC February newsletter 2024
The recent advancements in our ability to manipulate quantum mechanical systems are nothing short of astonishing. From controlling individual atoms, ions, and molecules to orchestrating interactions among multiple particles simultaneously, we now possess the capability to wield quantum phenomena with unprecedented precision. Yet, amidst these remarkable feats, the question arises: what practical applications can we derive from such mastery over quantum systems, and how can we fully unlock their potential?
Answering these questions is no simple task, primarily due to two key challenges. Firstly, we are still unraveling the precise characteristics that distinguish quantum mechanical systems from their classical counterparts. Secondly, the complexity inherent in controlling large quantum systems, particularly with imperfect technology, presents a formidable obstacle to scalability. In fact, we are not even able to provide a simple theoretical description behind the most common phenomena that are expected to happen in interacting quantum many-body systems: their thermalization. Therefore, the success of quantum science and technology largely hinges on improving our understanding of many-body quantum physics, a basic science question.
My research endeavors lie at the intersection of quantum many-body dynamics and quantum information science. My primary objective is to explore uncharted territories of many-body quantum physics and identify practical applications grounded in quantum principles. I pursue a multidisciplinary approach, combining theoretical, numerical, and experimental methods (in collaboration) to investigate exotic, far-from-equilibrium quantum dynamics, with a focus on devising applications in sensing, simulation, and information processing. Naturally, my research topics are spread across quantum information, condensed matter, and atomic molecular and optical physics. I believe that the best way to develop novel applications is to first better understand universal phenomena that occur in generic quantum systems. Consequently, a significant portion of my research endeavors is dedicated to addressing fundamental scientific inquiries such as quantum thermalization, chaos, its absence, and other intriguing dynamical phenomena.
In recent years, I have been particularly captivated by the potential of near-term quantum simulators. While not yet as sophisticated as full-fledged quantum computers, these simulators offer significant computational power and accuracy, enabling the exploration of otherwise inaccessible realms. However, leveraging these platforms presents its own set of challenges. Most existing quantum applications require applying fine-tuned control sequences to individual qubits separately and near perfectly, which is not feasible in many platforms.
In this newsletter, I am excited to share an alternative approach pioneered by my team at MIT. We focus on designing applications and protocols that require little to no quantum control, leveraging recent advances in quantum information and chaos theory, complemented by classical data processing techniques. Our key innovation lies in harnessing universal and emergent properties of chaotic quantum dynamics.
Measuring Arbitrary Physical Properties
For example, we’ve recently developed methods to efficiently extract arbitrary observables from analog quantum simulators, capitalizing on a universal phenomenon known as information scrambling. Conventional quantum simulation settings often limit the types of observables that can be directly measured within a device, such as particle or spin configurations in real space basis. However, we’ve recognized that in chaotic quantum dynamics, information about multiple observables becomes scrambled into a complicated form. In other words, by cleverly deciphering this scrambled information, aided by classical computation, we can deduce a multitude of observables from a simple set of measurements feasible in experiments. Building upon this insight, we’ve proposed an experimental protocol, where one intentionally scrambles observable information in a controlled manner, enabling the measurement of arbitrary observables, while only using existing or near-term technology.
Benchmarking Quantum Devices
Another avenue of our research exploits emergent properties of chaotic quantum wavefunctions, which manifest as distinctive statistical patterns known as “many-body speckles” upon measurement. These speckles offer unique signatures of quantum states, opening doors to practical applications. Our recent work focuses on leveraging quantum speckles to benchmark analog quantum simulators, specifically in estimating device fidelity. Our method, requiring minimal quantum control, has already been successfully implemented across two different platforms, enabling accurate characterization of their devices. Furthermore, we’ve extended this approach to quantify mixed-state entanglement in some of the largest analog quantum simulators with high fidelity.
Exploring Quantum Sensing
Currently, we’re delving into the extension of speckle-based applications to quantum sensing. We recognize the intimate relationship between quantum sensing and benchmarking – both aim to detect subtle changes in quantum dynamics. We approach these closely related problems with a fresh perspective. While benchmarking primarily focuses on overall performance evaluation, quantum sensing hones in on specific signals, demanding new methodologies, insights, and analysis.
In summary, our research endeavors, rooted in the intrinsic properties of chaotic quantum systems and augmented by classical data processing techniques, are aimed at advancing our quantum technology. Through collaborative efforts and multidisciplinary approaches, we strive to contribute to the ongoing exploration of quantum science and technology, with the hope of driving practical advancements in technology and making exciting new scientific discoveries.
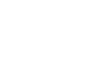

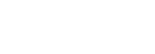

Copyright © 2022-2023 MIT Center for Quantum Engineering – all rights reserved – Accessibility