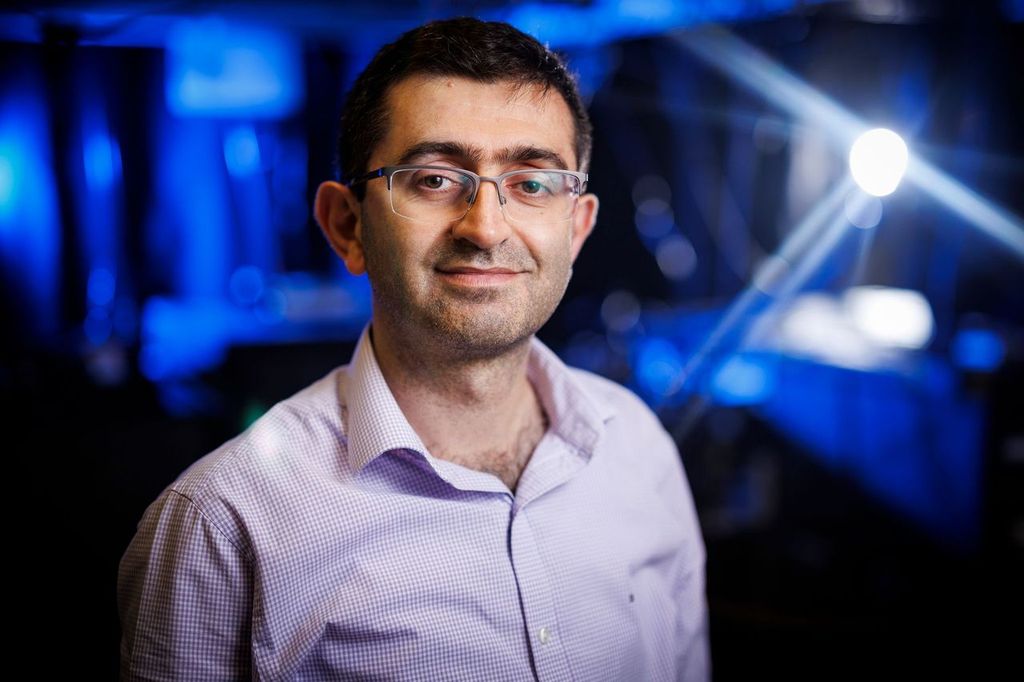
CQE PI Feature – Nuh Gedik
Probing and Controlling Quantum Materials with Light
Featured in QSEC February newsletter 2025
Quantum materials are compounds in which strong interactions give rise to fascinating phenomena, such as high-temperature superconductivity and colossal magnetoresistance. Despite decades of intense research, understanding the physics behind these materials using conventional techniques has proven extremely difficult. This is primarily because different degrees of freedom—charge, spin, and lattice—are strongly coupled in these systems, and the interplay between them drives their complex behavior.
The goal of my research is to uncover the physics of quantum materials by developing and applying novel time-resolved techniques. These methods allow us to create “movies” of excitations in these materials with femtosecond (10⁻¹⁵ second) time resolution. These movies help us disentangle the complex interactions governing the behavior of these systems. We have developed unique techniques capable of capturing different types of excitations—electronic, spin, or structural—with unprecedented time, energy, and momentum resolution.
Making movies with femtosecond time resolution is far beyond the capabilities of even the fastest conventional cameras. To overcome this challenge, we use ultrafast lasers that generate femtosecond light pulses. In a typical experiment, a single pulse is split into two: a stronger “pump” pulse excites the sample, while a weaker “probe” pulse measures the resulting changes—a technique known as pump-probe spectroscopy. By varying the time delay between the pump and probe pulses, we can construct a stroboscopic movie of the light-induced changes, achieving femtosecond time resolution without the need for a fast camera. We have also developed a range of probing methods to selectively measure specific properties, such as the energy and momentum of electrons, conductivity, or other optical constants.
Just as shaking a “black box” can reveal its internal structure, perturbing quantum materials with light and watching their response allows us to probe the complex interactions that govern them. At MIT, we have successfully applied these approaches to study a variety of quantum materials, including topological insulators (TIs), high-temperature superconductors, and atomically layered materials. Below, I highlight some recent advances where we not only use light to probe materials, but also to actively control their properties.
When an intense laser pulse strikes a material, the light is typically absorbed by electrons, creating an excited electronic state. However, when the light energy is tuned below the absorption threshold, novel quantum effects can emerge. In this regime, theory predicted that light could coherently hybridize with electronic states in the solid, forming new hybrid photon-electron states known as Floquet-Bloch (FB) states.
Although FB states had been theoretically proposed, they had never been observed in solids until we achieved their first experimental detection in topological insulators. We accomplished this by making femtosecond movies of the electronic energy levels while the material was illuminated with low-energy light. Using this technique, we successfully converted massless surface electrons in topological insulators into massive fermions. More broadly, this approach may enable switching a material’s phase—from conducting to insulating, or from transparent to opaque—simply by tuning the polarization, intensity, or frequency of the light.
Beyond topological insulators, the ability to manipulate electronic bands through coherent light-matter interaction holds great promise for many other systems. Recently, we directly observed FB states in graphene and applied similar methods to the thinnest known semiconducting crystals—monolayer transition-metal dichalcogenides (TMDs).
In TMDs, electronic energy levels feature two local minima, called “valleys.” There has been significant interest in exploiting these valleys to develop new types of devices capable of storing and processing information—an emerging field known as valleytronics. However, separating these valleys in energy normally requires extremely large magnetic fields due to their inherent symmetry. By illuminating TMDs with circularly polarized off-resonant light, we selectively shifted one of the valleys using the optical Stark effect. Our results offer a new way to manipulate the valley degree of freedom, which could pave the way for realizing novel Floquet topological phases in two-dimensional materials.
Controlling materials with light is not limited to coherent light-matter interactions. Another approach involves driving collective modes in a material to very high amplitudes, which can, under certain conditions, induce phase transitions.
We recently applied this method to an antiferromagnet—a material where neighboring spins align in opposite directions. Antiferromagnets hold significant promise for next-generation magnetic memory and information processing devices, but their weak response to external magnetic fields makes them difficult to control and manipulate. To overcome this, we used intense ultrashort terahertz (THz) pulses, with frequencies matched to the natural vibrational modes of the atoms in the material.
We found that ultrafast THz excitation of a layered antiferromagnet generated a new metastable magnetic state with a finite net magnetization. Remarkably, this state persisted for milliseconds even after the THz pulse ended. This phase transition can be understood by considering the coupling between atomic vibrations and magnetism. After THz excitation, atoms oscillate in a synchronized fashion at very large amplitudes, altering the interatomic distances. Since magnetic interactions depend strongly on atomic spacing, this structural response drives the observed magnetic phase transition. This approach could be extended to other materials, providing a powerful tool for controlling phase transitions with light.
Nuh Gedik joined the MIT Physics Department as an Assistant Professor in January 2008 and was promoted to Full Professor of Physics in 2018. He received his B.S. in Physics in 1998 from Bogazici University in Istanbul, Turkey, and his Ph.D. in Physics in 2004 from the University of California, Berkeley. Following his Ph.D., he worked as a postdoctoral scholar at Caltech until January 2008.
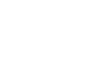

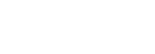

Copyright © 2022-2023 MIT Center for Quantum Engineering – all rights reserved – Accessibility