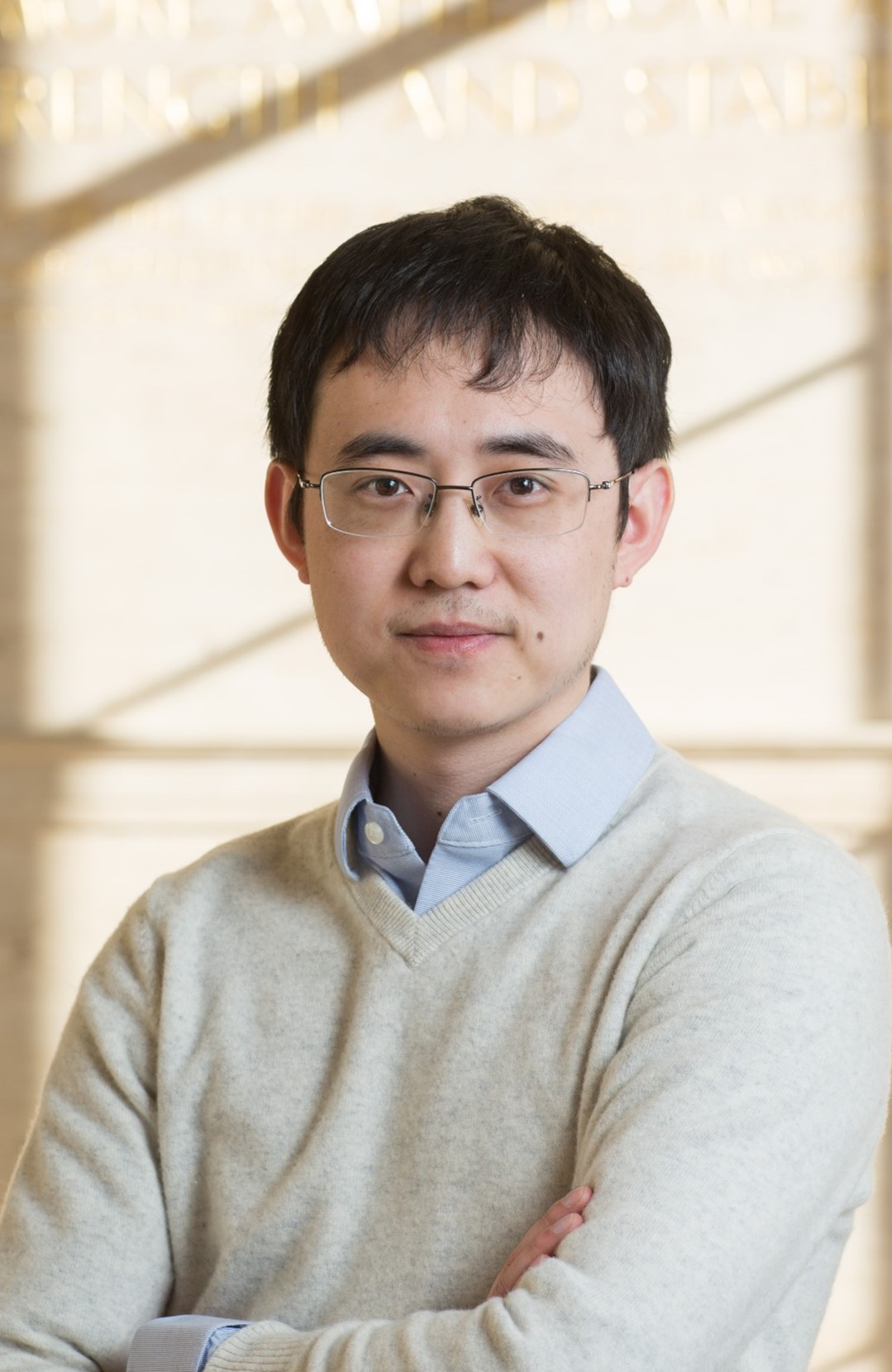
CQE PI Feature – Long Ju
Engineering Materials for Quantum Science and Engineering
Featured in QSEC October newsletter 2023
Quantum materials and quantum science are twins that entangle in many ways. The principles of the latter determines the behaviors of the former, and the former provides a platform to understand, engineer, and realize the applications of the latter.
As a condensed matter physicist, I study the properties of solid-state materials that are governed by the principles of quantum mechanics all the time. When vast numbers of atoms and electrons are put together in a crystalline form, their interactions result in emergent phenomena that is not possible when considering them as independent particles. Depending on the chemical composition and structure of crystals, such interactions can lead to a wide range of macroscopic states such as superconductivity, superfluidity as well as electron topology.
These states are so fascinating: why would these particles build coherence among themselves to behave as if they are one entity? Especially considering the inevitable existence of defects and disorders in solid-state materials? Can we engineer materials that host such effects at higher temperatures or even room temperature?
One such example is an orbital multiferroic state in pentalayer graphene that we reported recently. We know that electrons have an internal degree-of-freedom called spin. In materials like graphene, electrons have another degree-of-freedom called valley which characterizes their property in the momentum space. Similar to the spin pointing upward or downward, valley can also be thought of as an arrow that can preferably point to certain directions and can be switched by an effective ‘valley field’. We found that electrons in graphene can spontaneously polarize themselves in two ways at the same time: they can choose collectively to stay in the same valley in the momentum space, as well as align their magnetic moment in a specific direction in the real space. One can think of the whole graphene sample forming qubits in both senses of valley and magnetization, and they are coupled in a way to result in a total of four quantum states. Furthermore, one can use an electric field to switch between these four states to independently control the two internal degrees-of-freedom.
On the other hand, materials that exhibit such collective behaviors form the basis of applications in quantum science and engineering. Quantum computation usually suffers from the loss of coherence due to perturbation from the environment. In some schemes of quantum computation, the more robust property of solid-state materials called topology is introduced to fight against the perturbations and make the computation process more robust and reliable. One such example is the parafermion which was proposed to be realized by interfacing the fractional quantum Hall state with superconductivity. The fractional quantum Hall effect has been a paradigmatic phenomenon to exhibit the magic of electron correlation and topology, where the electrons are condensed to a state that has host fractional excitations: when the superposition of many unit charges is perturbed, the excitation behaves as if it has 1/3 (for example) of a unit charge! Such kind of states have been found when a big magnetic field is applied. But the dilemma of engineering parafermions lies in the fact that magnetic field usually kills superconductivity!
Through exploring the possible ground states of quantum materials, we recently found that one can have fractional quantum Hall effects in the absence of a magnetic field, again in pentalayer graphene. This discovery allows us to explore all the exciting physics of fractional quantum Hall states and superconductivity without worrying about the constraints set by big magnetic fields. We are very excited to explore the fractional excitations and the opportunities of engineering parafermions for topological quantum computation
I was lucky to join the field of two-dimensional (2D) quantum materials as a PhD at UC Berkeley. This field, although has been heavily explored since 20 years ago, still keeps inspiring us to study and draw an even bigger picture in the age of quantum science and technology. The big opportunities waiting for further investigations stem from the fact that you can design, construct and study completely unprecedented materials built out of individual 2D materials: they are like papers with different colors (properties); although being very different from their 3D counterparts and thus very interesting by themselves, the possibility of stacking papers together to create a mixed stack of papers is extremely intriguing. One can choose the colors, optimize the number of layers, and control the twist angle in these stacks to engineer the interactions between them—realizing quantum effects that were absent from any of the ingredient layers. The opportunities for quantum science and engineering in such a versatile platform has just begun to be revealed, and will promise for many years of intense studies.
At MIT, we work with other groups in the communities of quantum science and engineering. It is really important and exciting to share our own research with and learn from brightest people in the field on the most up-to-date developments. By integrating fundamental physics research of quantum materials and device engineering, we hope to establish new directions of research and develop solutions to problems met.
Long Ju joined the MIT Physics Department as an assistant professor in January 2019. He received his B.S. in Physics in 2009 from Tsinghua University, China, and his Ph.D. in Physics in 2015 from the University of California, Berkeley. He then moved to Cornell University, where he was a Kavli postdoctoral fellow until December 2018.
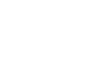

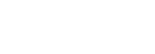

Copyright © 2022-2023 MIT Center for Quantum Engineering – all rights reserved – Accessibility