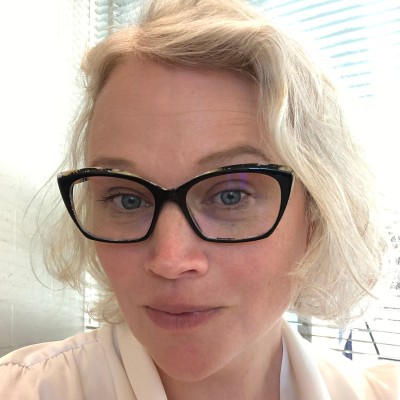
CQE PI Feature – Lindley Winslow
Featured in QSEC March newsletter 2025
Lindley Winslow is an experimental particle and nuclear physicist whose research seeks to understand how the universe’s smallest constituents shaped the cosmos we observe today.
“I love thinking about the big questions that are at their heart a question of why did we get so lucky to exist.”
Her work focuses on precision measurements in the search for axion dark matter and neutrinoless double-beta decay—two phenomena that lie at the heart of the universe’s most fundamental mysteries.
Dark matter, which makes up 85% of the matter in the universe, does not emit or interact with light. Despite its overwhelming presence, we still don’t know what it is. Axion dark matter experiments aim to shed light on this invisible component of the universe. Axions are hypothetical particles originally proposed to solve a puzzle in quantum chromodynamics, but they also happen to be a leading candidate for dark matter—light, weakly interacting particles that may have been abundantly produced in the early universe.
Neutrinoless double-beta decay probes an even deeper mystery: why the universe is made of matter at all. According to known physics, all processes should produce equal amounts of matter and antimatter—yet we live in a universe dominated by matter. The discovery of neutrinoless double-beta decay would reveal a process that violates this symmetry. It would also confirm that neutrinos are Majorana particles—meaning they are their own antiparticles—profoundly reshaping our understanding of lepton number conservation and the role of neutrinos in the early universe.
“For an experimentalist, these questions not only address interesting science but also challenge us to push the bounds of what is measurable—whether it be minuscule deviations in electricity and magnetism or finding one decay per year in a ton of isotope.”
A revolution in quantum sensing is driving advances in both research directions. Axion experiments demand techniques that push beyond quantum mechanical limits, while double-beta decay experiments require the integration of quantum-limited devices across cubic-meter-scale arrays of ultra-pure crystals.
“Quantum sensors have opened up new measurements that we never thought were possible, and the physics presents new challenges in scaling and integration… challenges that connect us to other interesting engineering problems.”
The engineering demands are as ambitious as the physics goals. Double-beta decay experiments require the growth of high-purity crystals and the design of cabling and sensor systems that meet strict radio-purity and thermal constraints. For axion searches, a single ultra-sensitive sensor must be coupled to a high-Q resonator operating in the kilohertz regime—all cooled to millikelvin temperatures—within a large, high-field magnet on par with those developed for fusion research.
The intersecting challenges in quantum sensing, crystal growth, cryogenics, and magnet technology make these some of the most complex experiments in physics today—but also among the most exciting.
“The universe doesn’t give up its secrets easily, and we’re going to have a lot of fun tackling these engineering challenges as we try to discover them.”
Tackling these fundamental questions requires not only technical innovation but also strong collaboration across institutions and disciplines. We are always looking to broaden our network of collaborators—whether in quantum sensing, cryogenics, crystal growth, materials science, or theoretical modeling. These problems are too big for any one group to solve alone, and we welcome new ideas and partnerships that help push the boundaries of what’s possible in the search for dark matter and the origin of the universe’s matter.
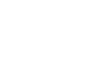

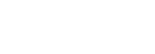

Copyright © 2022-2023 MIT Center for Quantum Engineering – all rights reserved – Accessibility