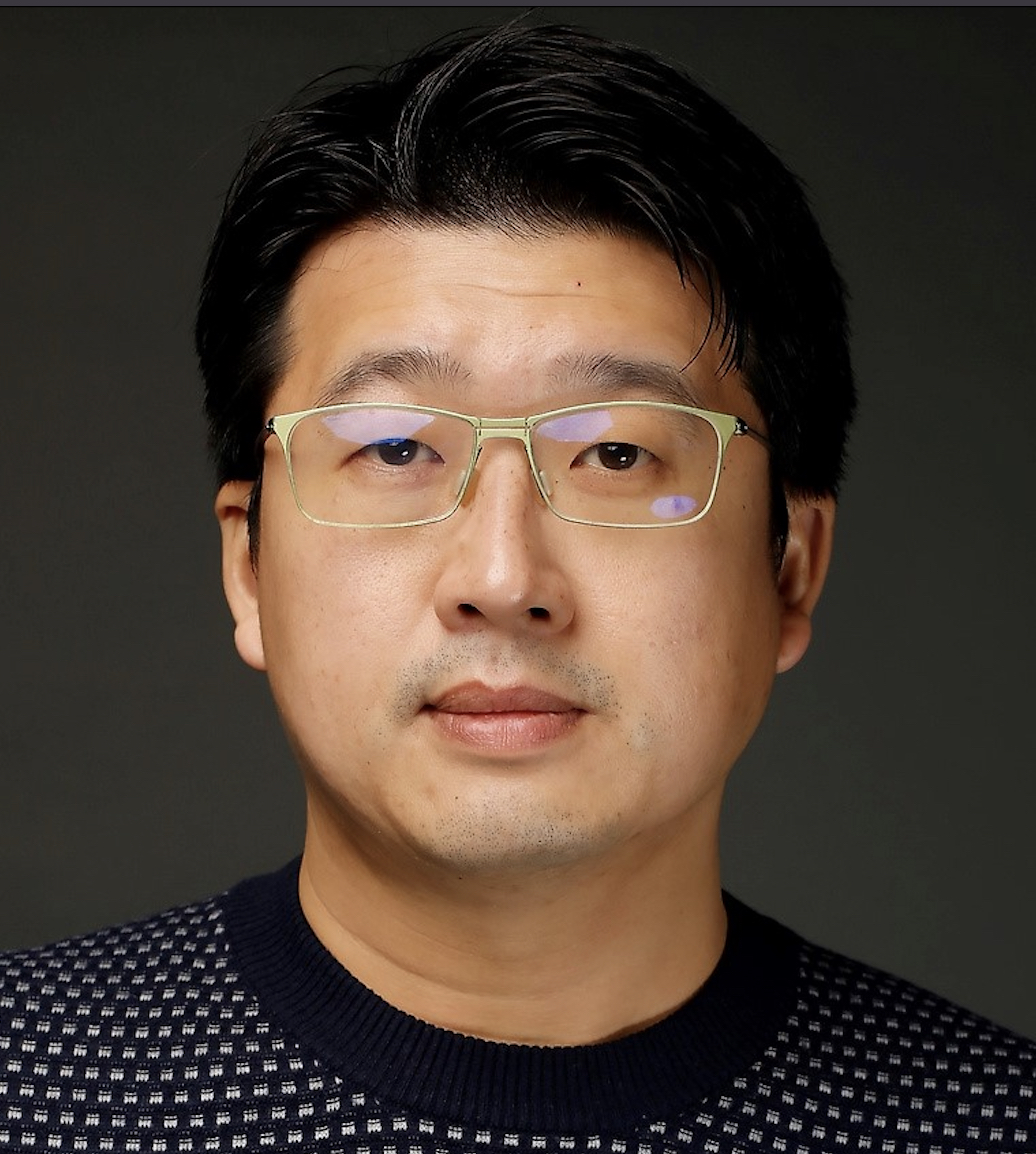
CQE PI Feature – Joel I-jian Wang
Featured in QSEC August newsletter 2024
A qubit is sometimes referred to as an artificial atom due to its discrete energy levels, which can be externally addressed. Just as a natural atom consists of protons, neutrons, and electrons, an artificial atom can be constructed using electronic components such as capacitors, inductors, and Josephson junctions made from superconducting materials. The family of superconducting qubits is built on this concept, and the qubit’s characteristics can be engineered by incorporating additional superconducting elements into the device.
I’ve always been fascinated by the fact that quantum objects can emerge from the collective behavior of macroscopic electronic components. This opens up pathways not only to enhance the performance of superconducting qubits but also to create new quantum devices by leveraging the properties of novel materials systems.
The materials systems we focus on are van der Waals materials and their heterostructures.
Van der Waals materials consist of a variety of layered 2D crystals, spanning semi-metals, insulators, semiconductors, ferromagnets, superconductors, and topological insulators. These materials can be assembled in nearly any desired order to form van der Waals heterostructures for different applications. The pristine, atomically flat interfaces and crystallinity of these layered materials offer significant potential for constructing high-quality components for emerging solid-state quantum computing platforms.
My scientific exploration at the intersection of 2D materials and superconducting quantum technology began with a collaboration with EQuS, led by Professor Will Oliver, while I was a graduate student in Professor Pablo Jarillo-Herrero’s group. Together, we demonstrated the first voltage-tunable superconducting qubit made with graphene, which initiated my journey into studying 2D materials using circuit quantum electrodynamics (cQED).
At EQuS, we leverage the unique properties of 2D materials to enhance or complement superconducting quantum technologies, with the goal of enabling extensible solid-state quantum computing platforms in the future. For instance, 2D insulators such as hBN or TMD semiconductors can offer high-quality dielectrics for creating Josephson junctions or shunting capacitors with low microwave loss. These materials can also be combined with 2D superconductors to construct lump-element devices or even qubits entirely from van der Waals materials, significantly reducing footprint or suppressing cross-talk between neighboring qubits due to the distributed microwave field. Additionally, 2D superconductors, which retain their superconducting properties down to a single layer of crystal, may provide high kinetic inductance, enabling the creation of inductors for certain types of superconducting qubits, such as fluxonium or zero-pi qubits, or for quantum sensors.
Conversely, superconducting circuits offer a unique toolkit for investigating novel quantum materials, complementing traditional quantum transport measurements. For example, the inductive response of a superconductor provides insight into Cooper pair density, or more generally, superfluid stiffness, which determines a superconductor’s electromagnetic response. The capacitive response of quantum materials can reveal critical information about their density of states or band structures. High-frequency probes (up to tens of GHz) make it possible to study non-equilibrium behavior and other dynamic processes in novel materials using time-domain techniques. These characterizations are significantly enhanced by the cQED platforms developed for qubit control and readout.
Superconducting quantum technology and quantum materials are vast, vibrant, and exciting research fields. Working at their intersection fulfills my curiosity and passion in both directions. However, this journey is only possible because of the privilege of collaborating with talented postdocs, graduate students, and UROPs at EQuS, who share this enthusiasm for exploration.
At EQuS, our collaborative environment drives progress. The exchange of ideas among group members not only enriches the scientific process but also sparks creativity. By fostering a balanced culture of work and life, we ensure that our team stays energized and motivated, which is essential for sustained productivity and innovation.
Beyond the intellectual excitement, our research thrives on the synergy between disciplines fostered by the collaborative culture at MIT CQE and EQuS, as well as our close partnerships with colleagues at MIT Lincoln Laboratory. The diversity of ideas and expertise continually drives us to overcome experimental challenges and push the boundaries of what is possible.
Our coffee machine—and the speed we go through beans—can certainly attest to it.
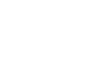

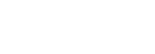

Copyright © 2022-2023 MIT Center for Quantum Engineering – all rights reserved – Accessibility