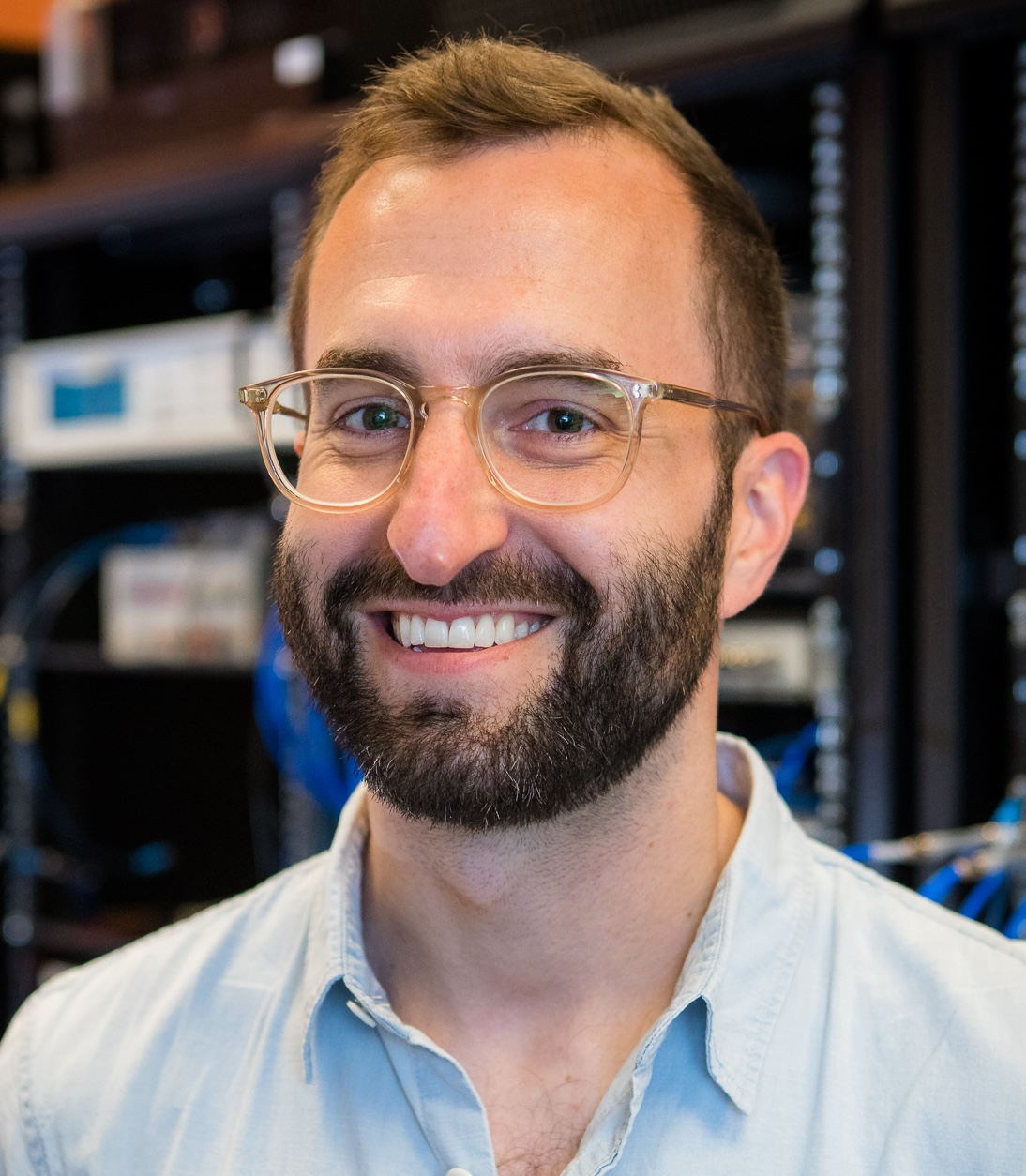
CQE PI Feature – Jeffrey Grover
Featured in QSEC December newsletter 2023
Much of the research I do today is the result of returning to my past, often serendipitously. As an undergrad 15 years ago, my first foray into physics research was a senior honors thesis. There were contemporaneous, beautiful experiments from MIT and MIT Lincoln Laboratory that measured what happens when you take a two-level quantum system—in this case, a superconducting flux qubit—and repeatedly traverse its anticrossing. They observed interference patterns that could be experimentally controlled, and we explored their algorithmic utility, e.g. for prime factorization. This project was not scientifically successful, but it spurred some important personal discoveries. First, I am not a theorist. And second, I became passionate about doing quantum science. The happy coincidence of this story is that I am now a Research Scientist in the same group at MIT that did those experiments, the Engineering Quantum Systems Group (EQuS) led by Professors Will Oliver and Terry Orlando.
In EQuS, we perform experimental quantum information science using superconducting circuits as our modality of choice. These are macroscopic devices that can behave quantum-mechanically, and we can precisely tailor their parameters via design and fabrication. We design them such that they behave like artificial atoms, with clearly addressable levels for quantum computing applications.
We study superconducting qubits from a variety of angles and at different levels of scale. For instance, we improve the coherence of individual qubits by improving the underlying materials or by designing them such that they are protected by pernicious sources of noise. We also push 1- and 2-qubit fidelities to state-of-the-art values by using new couplers or by implementing novel control protocols. At the larger end of the spectrum, we use arrays of 16+ coupled qubits to perform programmable quantum simulation, which many experts feel will be the application area with the shortest path to real-world impact. This list doesn’t cover all the projects happening in our group, and that breadth is something I find particularly exciting about EQuS—and MIT’s quantum research in general.
I have a particular affinity for our experiments with connections to those in atomic physics and quantum optics. One platform that falls into this paradigm is called waveguide quantum electrodynamics, waveguide QED for short. In waveguide QED, we couple an atom—artificial or natural—to a waveguide to engender strong atom-photon interactions. To achieve the desired strength, we want the mode area of the waveguide to be small, and we want the cross-section of the atom to be large: this creates a large overlap between the atom and the photonic mode so that they can coherently exchange information. For my PhD, we built waveguide QED systems by coupling cold rubidium atoms to an optical nanofiber. An optical nanofiber is regular optical fiber (think Version FiOS) stretched thin to the point where its diameter is less than the wavelength of light propagating through it. This results in an intense evanescent field where a large fraction of the light is outside the fiber, creating a region where atoms can strongly interact with photons. We achieved coupling efficiencies of about 10%, which is impressive for real atoms and optical photons—but there is another way! Fast-forward 7 years to EQuS, where we make similar systems with artificial atoms—superconducting qubits—coupled to microwave waveguides. Given the designability our qubits, we can increase their effective cross-section relative to the mode area of the waveguide, such that coupling efficiencies of 99.9% are readily achievable. Moreover, we can do fun things like couple the same qubit to multiple points of a waveguide, or tunably change the effective distance between neighboring qubits. This toolbox has enabled some fun experiments in the lab like generating entangled photons or creating so-called giant atoms. Most recently we implemented a module that can deterministically send a single photon in a chosen direction: this could be a building block for a quantum interconnect within a larger processor.
The work I described above has an important common ingredient: software. As we add more qubits to our systems, the number of control knobs grows in kind. This increases the complexity of tune-up, calibration, and measurement, necessitating software that can handle that complexity. Before joining EQuS, I did a stint as a data scientist for a healthcare software company. What struck me about software development in the real world is that 95% of the work is not inventing clever algorithms with better scaling. Rather it’s building software that’s well-documented, well-tested, and easy to generalize. Given the nature of academic research, this is impossible to perfectly emulate, nor is it necessarily desirable: it takes a lot of effort that would distract from doing science. However, I try to find a middle ground that applies some best practices to our work so that it facilitates the cutting-edge research we want to do. I also hope to imbue the students with these development philosophies so that they have a useful skill to carry with them after they leave MIT.
Quantum computing research is interesting, but that alone can’t sustain a career. Thankfully, EQuS possesses an incredible culture that is unlike any I have experienced in academia. The deepest joy I get from this work is from helping to nurture that culture. I want to show students that doing world-leading research doesn’t require that they sacrifice a collaborative and supportive work environment, but rather that it is exactly this sort of environment that enables the research.
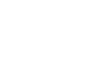

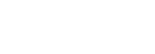

Copyright © 2022-2023 MIT Center for Quantum Engineering – all rights reserved – Accessibility