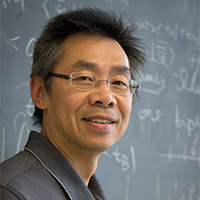
CQE PI Feature – Franco N.C. Wong
Featured in QSEC December newsletter 2024
My first introduction to quantum optics was a graduate course taught by Joe Eberly at the University of Rochester where I was an undergraduate. I learned about interactions of light with an atomic ensemble which lead to a variety of phenomena such as free induction decay and photon echo. I was fascinated with the simplicity and elegance of the theoretical approach. Nowadays we consider that as semi-classical optics in which the quantization of the optical field is not necessary, not until later when squeezing and entanglement emerged as the mainstay of quantum optics. That course led to my first publication when I spent a summer doing research with Joe Eberly, as well as to my graduate-school choice of a warmer locale at Stanford University. My thesis work at Stanford was actually done at IBM Almaden with Dick Brewer on coherent optical detection of nuclear spin effects in low-temperature Pr3+:LaF3 (praseodymium in lanthanum trifluoride) with extremely narrow kilohertz optical linewidths. More recently, the same system is potentially useful for qubit storage with long memory lifetimes provided by the nuclear spins and it has an enormous storage spectral capacity due to its very broad inhomogeneous linewidth of many gigahertz. I certainly had no idea then what it was good for, but it reinforces the adage that basic research studies may yet find their applications (often decades later).
After a postdoc study at the Joint Institute of Laboratory Astrophysics (JILA) with Jan Hall, arguably the world’s top expert on ultrahigh precision measurements and feedback control techniques, I joined Jeff Shapiro’s group in 1983 to begin my MIT journey of scientific exploration. A critical lesson I learned at JILA is that noise is something that one can tame using the proper feedback technique and that one should avoid working in a noisy regime (spectrally, for example) in order to get higher signal-to-noise levels, which of course applies to everything we do in science. While the lesson seems quite obvious, I have found that some scientists, especially those who have very little exposure to feedback control, are afraid of working with setups that incorporate cavity stabilization and servo loops. It has been an advantage for me by allowing me to make first attempts at systems (with servo control) that others are hesitant to try.
During my early MIT years, I dabbled in precision measurements by applying my work in optical squeezing to methods of relating microwave frequencies to optical frequencies. I came up with the idea of using optical parametric oscillators as a way to perform 2-to-1 as well as 3-to-1 optical frequency division, both of which we demonstrated experimentally, and we devised a scheme to link optical frequency standards to the cesium microwave frequency standard. Alas, my scheme was too cumbersome and it was no match to the scheme adopted worldwide using a wideband octave-spanning frequency comb that was invented and demonstrated by my two former mentors, Jan Hall and Ted Hänsch.
My focus at MIT has always been the study of the quantum nature of light and my collaboration with Jeff has been complementary in terms of theory and experiment. His expertise has helped me understand and navigate often confusing experimental choices. We initially focused on quadrature squeezing with an emphasis on sub-shot-noise detection, but we eventually settled on entanglement generation and its many potential applications. At the time, the general method of generating polarization entanglement between two photons was to create two directionally non-orthogonal cones of light, one cone for one photon species and the other for the conjugate photon species. These two cones of light intersect at two points, at which polarization entanglement can be observed. My background in nonlinear optics informed me that scheme was not efficient. Subsequently, we engineered the system by collapsing the two cones and making them overlap such that the outputs were beam-like and all the photons were useful instead of just those at two intersecting points. This provided a big step forward in entanglement generation and these high-flux devices could be utilized in applications. Another big leap forward was the realization of a Sagnac interferometric setup that provides high flux, high polarization entanglement as well as automatic phase compensation. One interesting highlight of this Sagnac source is that it was the basis for the first photonic entanglement source ever flown in space in a Chinese satellite (reported in 2017).
The Shapiro-Wong group derived some satisfaction from my occasional obsession in achieving “perfection” by experimentally reaching the theoretically maximum limits. In the process, we were able to solve or at least bypass various previously unknown obstacles. For the Sagnac entanglement source we achieved the Clauser-Horne-Shimony-Holt inequality limit of 2√2, for nonlocal Franson interferometry a visibility of unity, and for pure-state single photons a very high purity (all within small experimental uncertainties, of course). What made achieving these high-quality measurements possible were the theoretical support that Jeff often supplied with clarity as well as the students and postdocs who performed the experiments with steadfast focus and dedication. Without them, the foray into quantum-based communication, sensing, and key distribution, single-photon imaging, and, most recently, quantum frequency conversion between quantum-memory wavelengths in the near-IR and telecom wavelengths (for connecting remote nodes of a quantum network) would have been impossible.
Quantum research never ceases to amaze me no matter how many years I have been working in the area. When confronted with a new scheme, it can be tricky to quickly decide how it works and whether it is quantum or just classical physics cloaked in a fake-quantum façade. Jeff and I have fond memories of a presentation we made some years ago of our classical measurements to an audience of quantum physicists. A chorus of them kept suggesting that what we saw could be due to some quantum phenomenon, despite our insistence that our theory and experiment were really classical. On another occasion, I was excited to tell a sponsor that I could achieve the same quantum results with classical methods and with much higher flux, only to be told that he was not interested in anything classical! Such are unusual encounters in the life of a quantum physicist (or engineer).
My favorite playful-but-true saying to visitors or conference audiences on quantum entanglement is that: the less we know, the better the entanglement source is, and that if we know nothing, the entanglement source is perfect! In reality this is often the case because in order to generate high quality entanglement it is necessary to erase any distinguishing information about what’s going on. Conversely, if we know everything, then no entanglement remains and indeed such is the case for our generation of pure-state single photons.
My interactions with students and postdocs are what make doing research at MIT and RLE so engaging and rewarding. I have often learned from senior graduate students and seasoned postdocs who made valuable suggestions to experimental setups and measurement techniques that have typically led to fantastic results. As I approach the time to retire in the very near future, I will miss all the exciting interactions with PIs, postdocs, and students, as well as the RLE staff who has provided me with remarkable support. I will certainly keep track of all the amazing accomplishments in the quantum field at MIT and elsewhere. Maybe I will see in my lifetime a true quantum computer doing real applications over a quantum network with many qubits and error correction protocols. I feel that quantum research is a long-term growth area for research, funding, and people investment, especially for the younger generation.
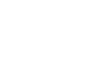

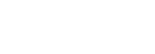

Copyright © 2022-2023 MIT Center for Quantum Engineering – all rights reserved – Accessibility